Introduction to Rotary Airlock Valve
Introduction to Rotary Airlock Valve (RAV)
Pneumatic conveying systems play a vital role in efficiently transporting materials across various industries. A key player in these systems is the rotary airlock valve. If you’re unfamiliar with what rotary airlock valves are, how they operate, the various types available, and their typical applications, we’ve got you covered. This article aims to provide all the essential information about rotary airlock valves, commonly referred to as RAV, so you can gain a comprehensive understanding of their functionality and importance in material transport systems.
What is Rotary Airlock Valve?
Rotary airlocks go by various names like rotary locks, rotary feeders, or simply “airlocks.” Despite the different terms, their main job stays the same. They make it easy to move dry, free-flowing bulk solids—like powders, granules, pellets, nuggets, dust, and crystals—from a storage bin, storage silo, hopper, cyclone, or receiver through a pneumatic conveying system to the next step in the manufacturing process. These valves are essential for keeping material handling and pneumatic conveying systems efficient and reliable. They ensure a controlled and uninterrupted flow of bulk materials, prevent air leakage, and maintain system pressure.
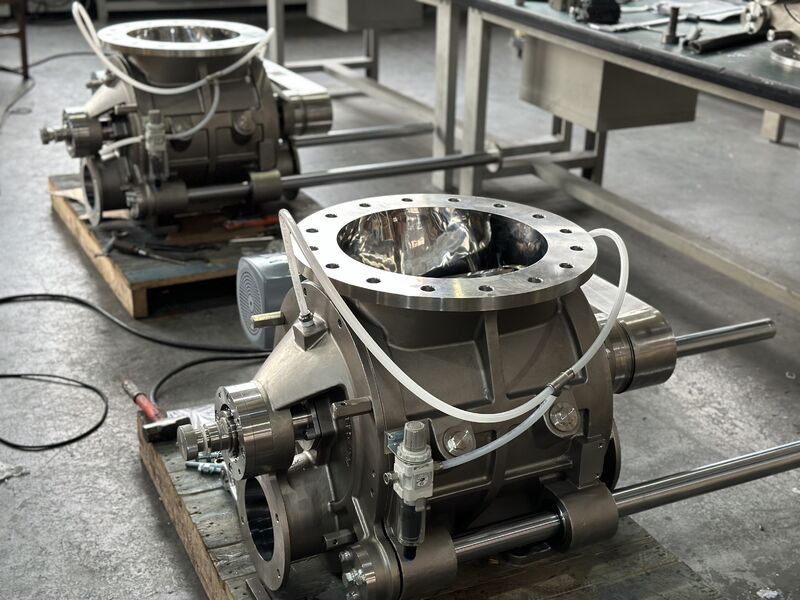
How Does a Rotary Airlock Valve Work?
A rotary airlock is comprised of several key components, including a casted outer housing, a fabricated multi-vane rotor and shaft, endplates, a drive, shaft packing seals, and bearings.
Here’s a breakdown of its operation:
❖ Functionality:
- When activated, the vanes of the rotary airlock rotate within the housing, resembling the movement of a revolving door. The primary objective is to facilitate the controlled movement of materials while preventing pressure loss, much like a revolving door manages the flow of people while preserving the indoor environment.
❖ Rotor Pocket:
- The space between the rotating vanes forms the rotor pocket. This is where incoming material enters from the top opening and stays before being pushed out through the lower opening, continuing its journey through the pneumatic lines.
❖ Pressure Control:
- The tight clearance between the vanes and housing minimizes pressure loss in the system. This is crucial, especially when dealing with varying pressures between bins, silos, hoppers, and the adjacent operation. Placing airlocks at the beginning of a pressure system or at the end of a vacuum system helps maintain optimal pressure conditions.
Types of Rotary Airlock Valves
Rotary airlock valves come in various types, each designed to suit specific applications and operational requirements. Here are some common types of rotary airlock valves:
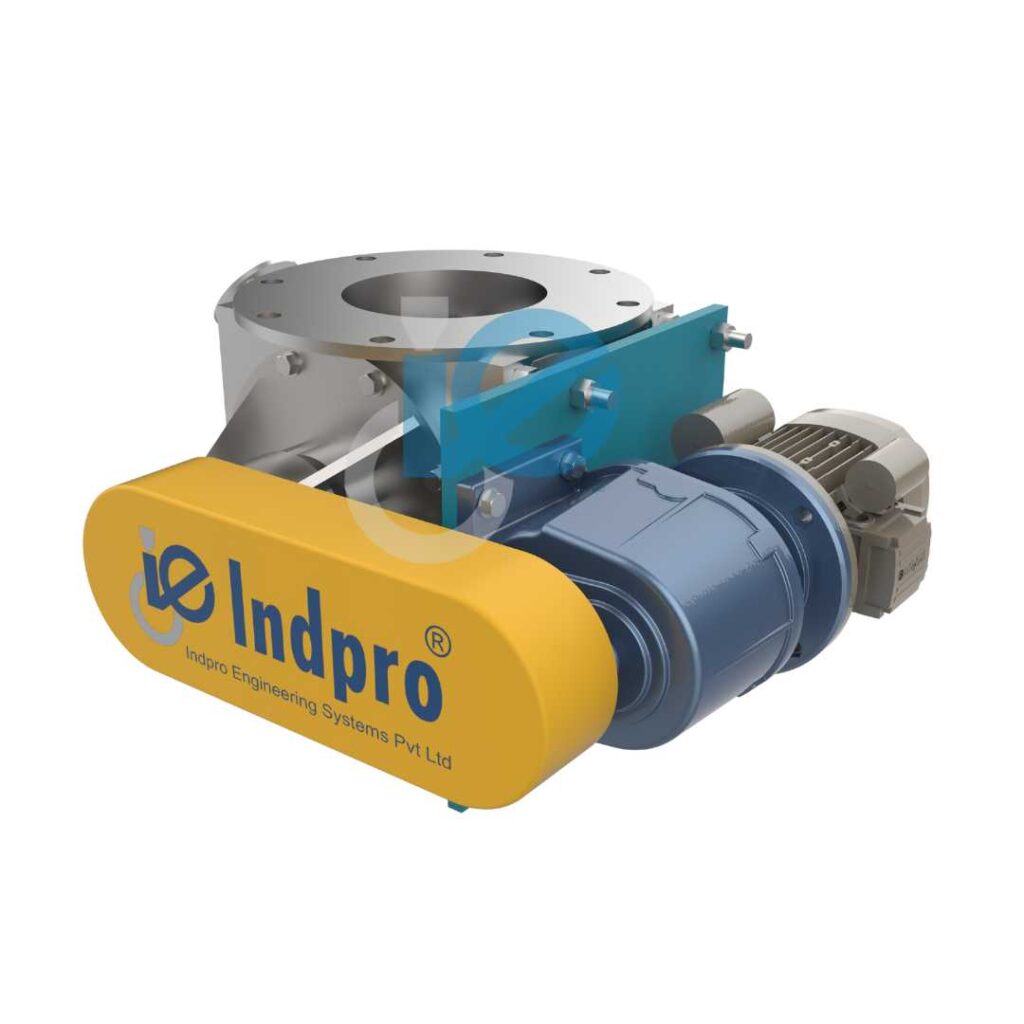
Fall-Through Rotary Airlock Valve:
- In this type, the material drops through the valve and is discharged at the bottom.
- It is suitable for gravity-fed applications where the material flows directly through the valve.
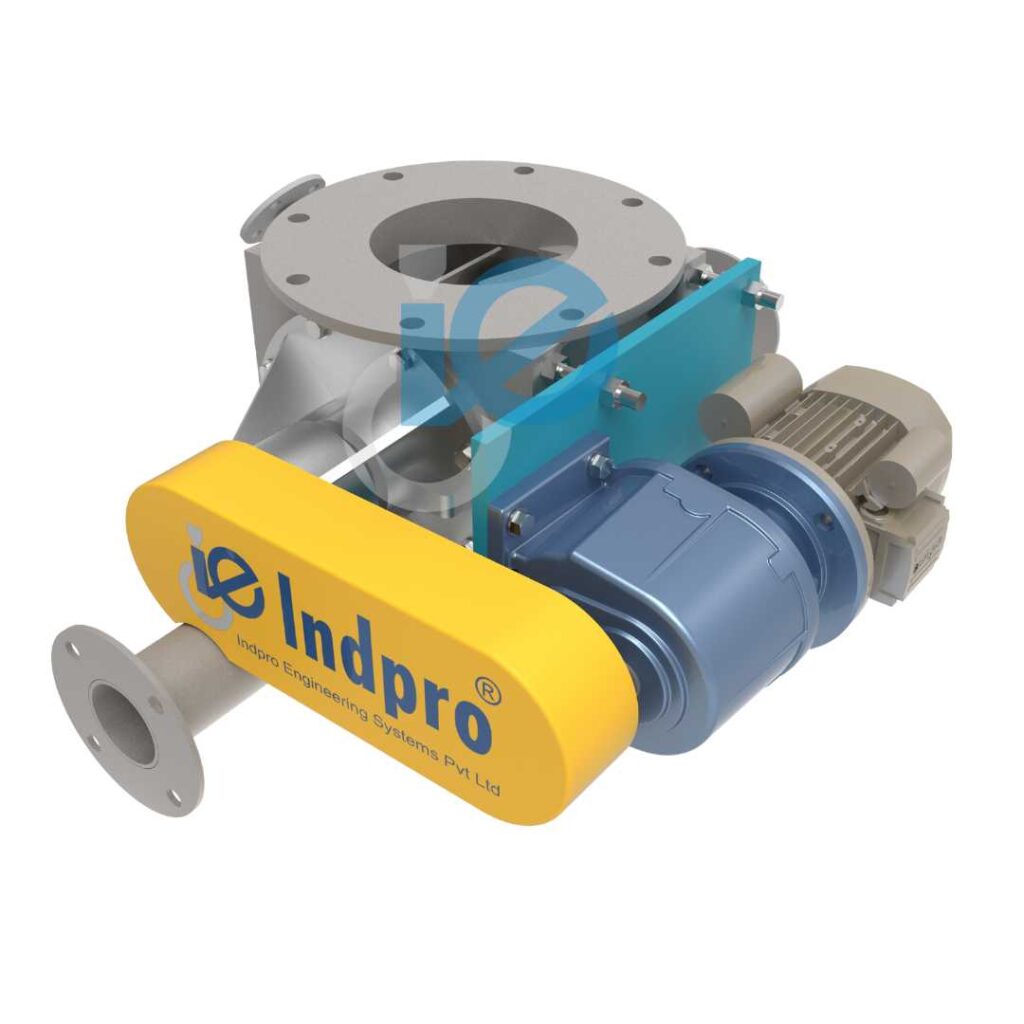
Blow-Through Rotary Airlock Valve:
- Also known as “conveying” or “pneumatic” rotary valve.
- Designed for use in pneumatic conveying systems.
- The material is blown through the valve with the assistance of an air or gas stream.
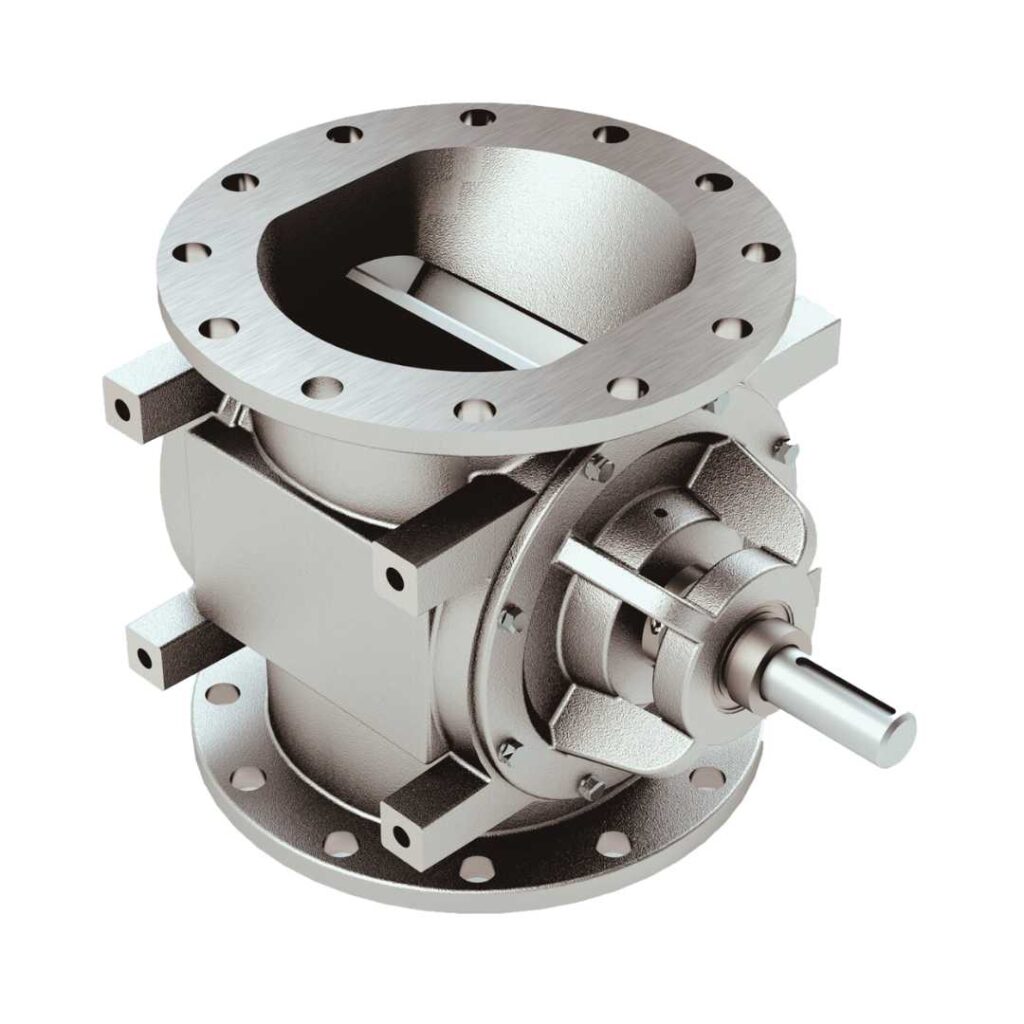
Standard Duty Rotary Airlock Valve:
- Commonly used for general-purpose applications where standard pressure differentials and material characteristics are present.
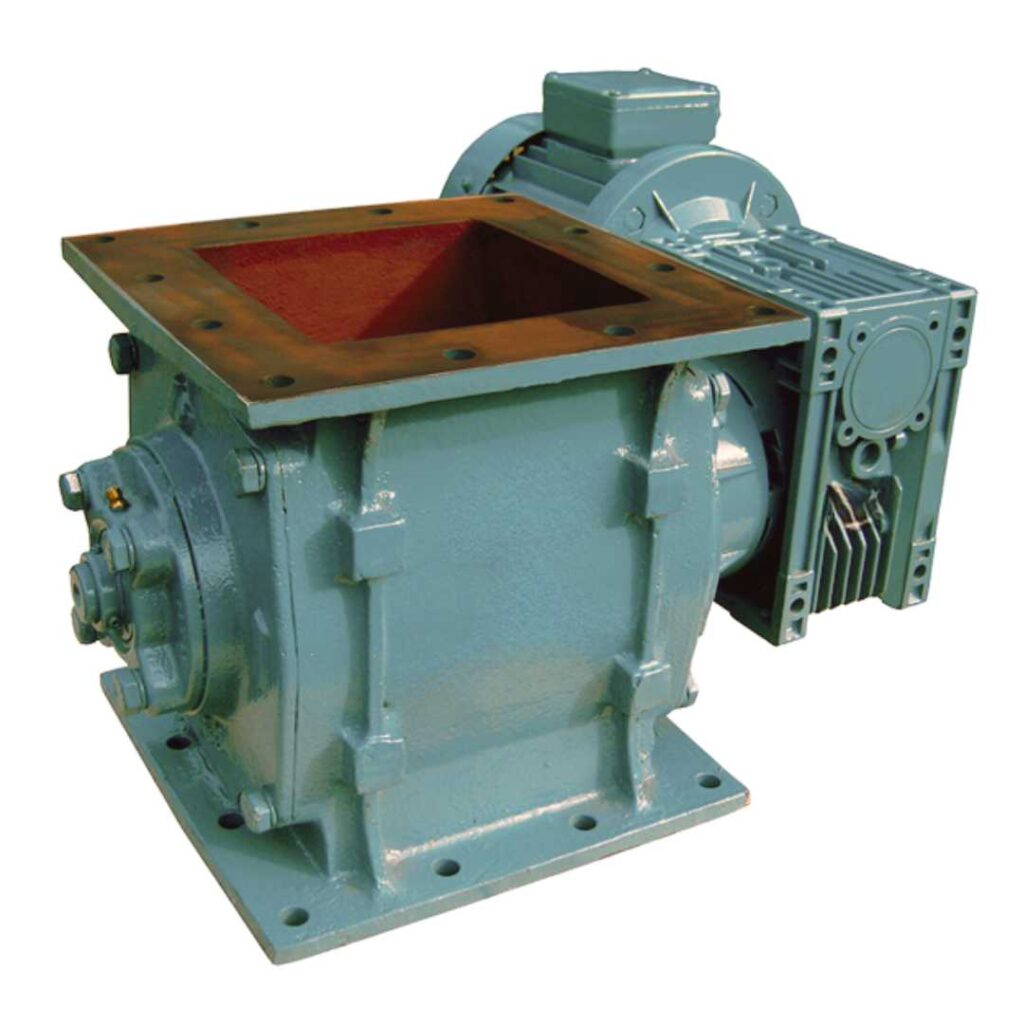
Heavy Duty Rotary Airlock Valve:
- Designed for handling more abrasive or challenging materials.
- Features robust construction to withstand wear and tear in demanding environments.
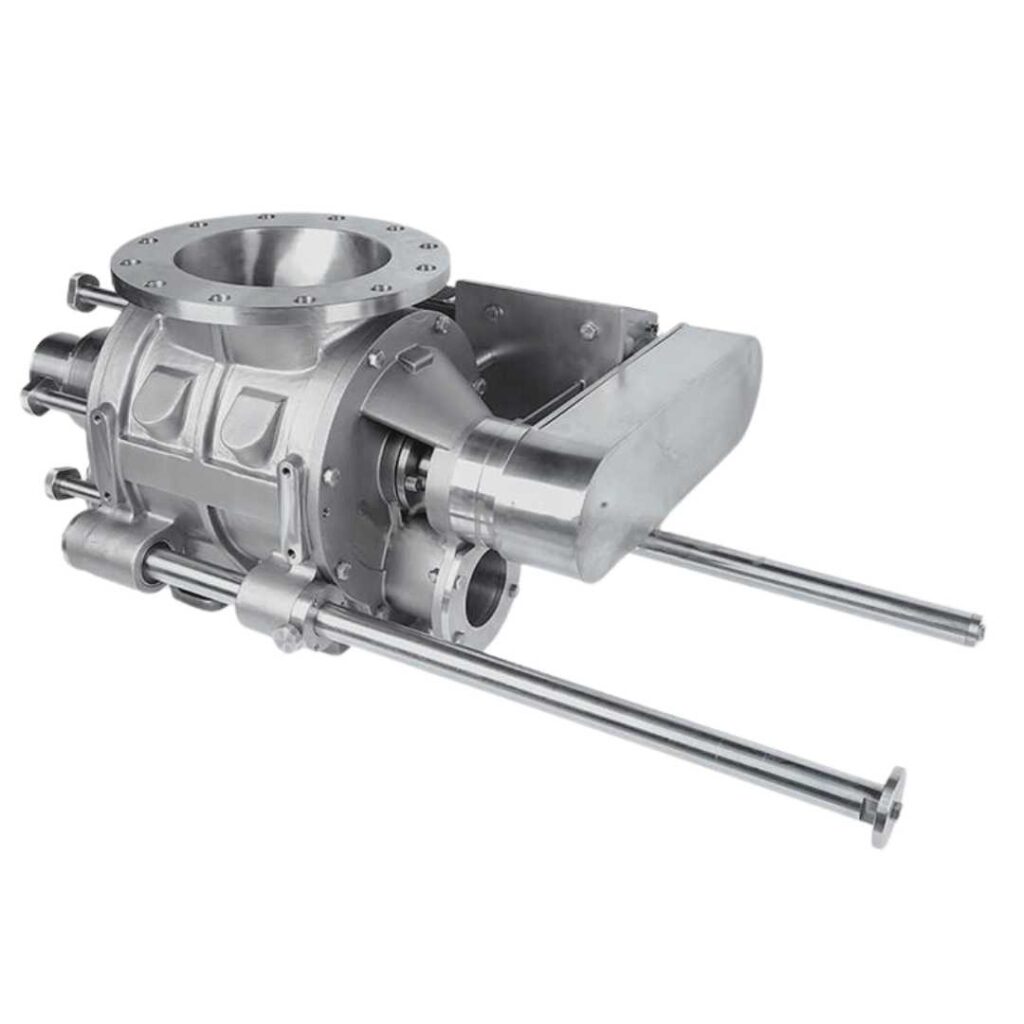
Quick-Clean Rotary Airlock Valve:
- Designed for easy and quick disassembly and cleaning.
- Suitable for applications where frequent cleaning and sanitation are necessary.
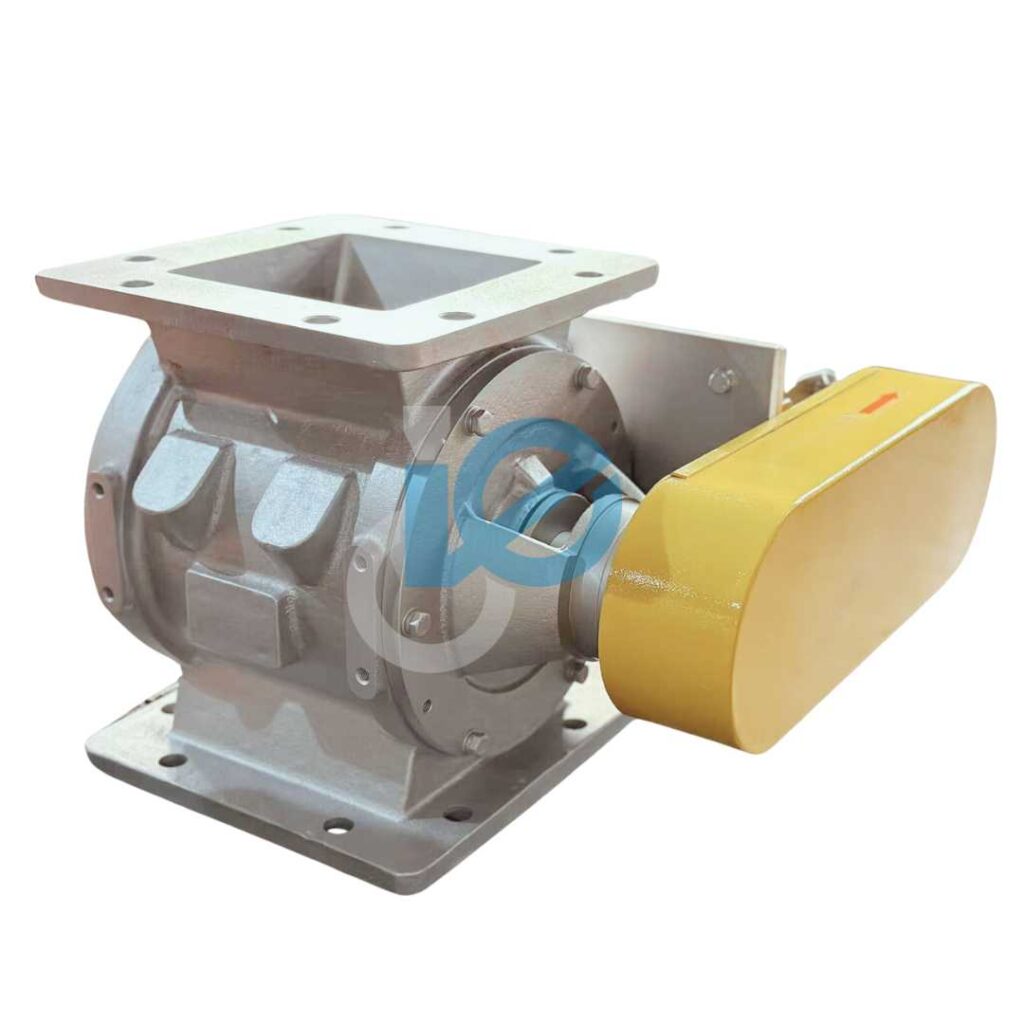
Dust Collector (Dust Disposal) Rotary Airlock Valve:
- Especially used in dust collection systems.
- Helps maintain a seal and prevent dust from escaping the collection system.
Seals used in Rotary Airlock Valve
Rotary airlock valves use various types of seals to maintain an airtight or dust-tight seal between different pressure zones in material handling and processing systems. Here are common types of seals used in rotary airlock valves:
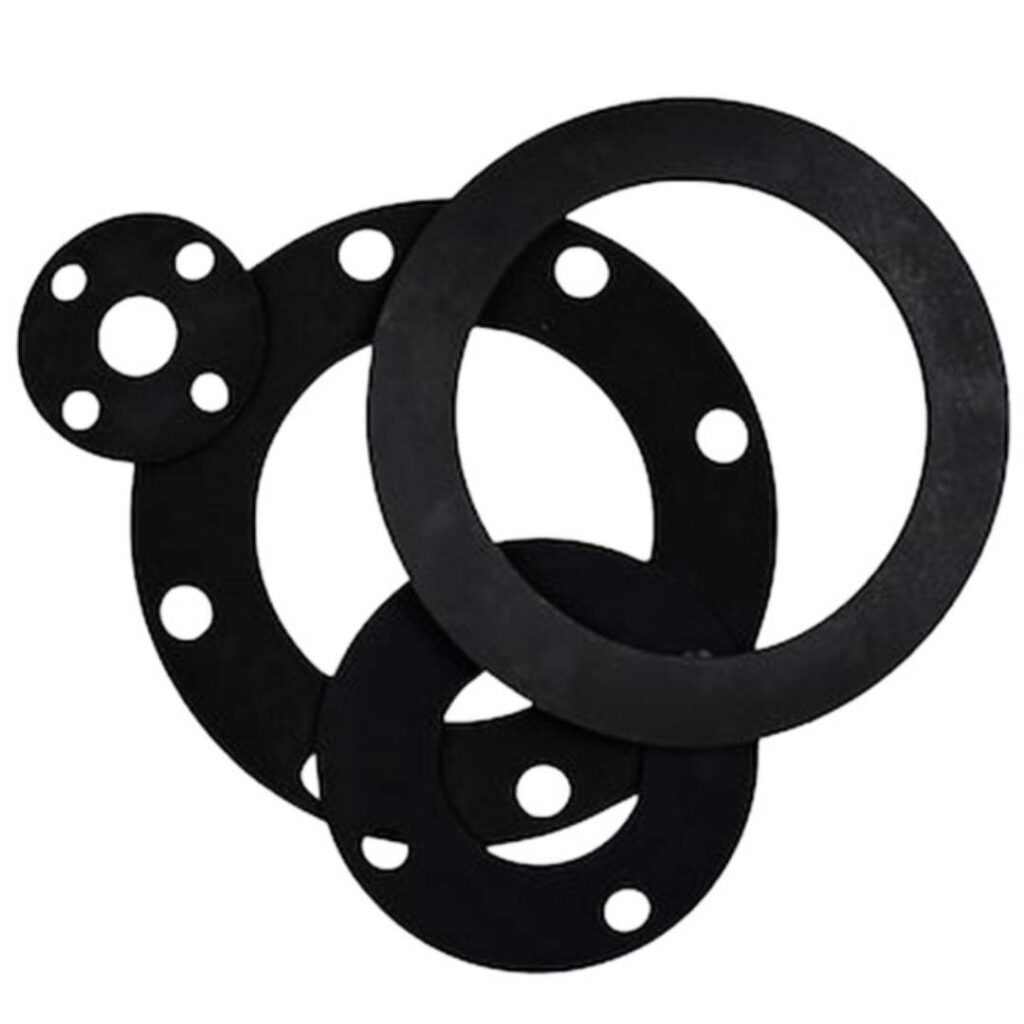
Flexible Rubber Seals:
- Soft rubber seals are commonly used for their flexibility and ability to conform to irregularities on the rotor and housing surfaces.
- They provide an effective seal and are suitable for general-purpose applications.
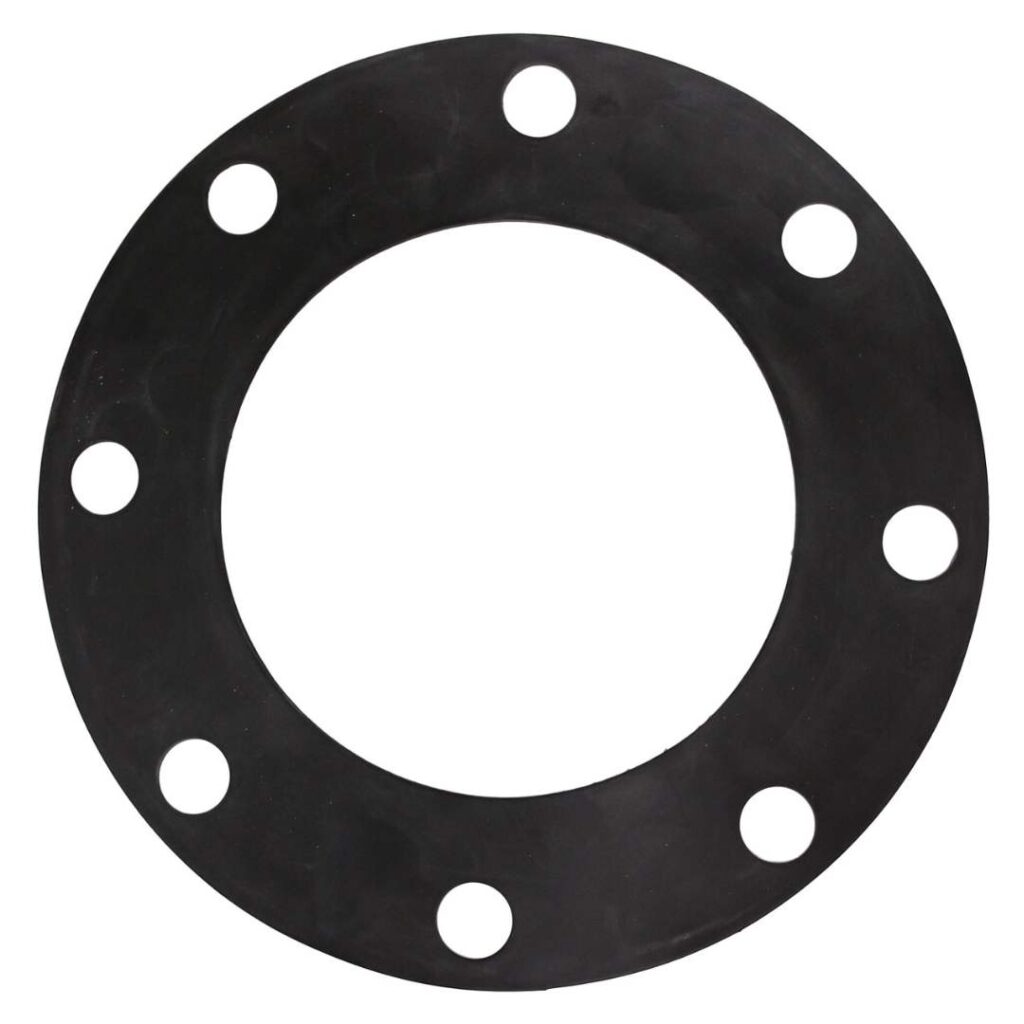
Neoprene Seals:
- Neoprene is a type of synthetic rubber known for its durability and resistance to abrasion, weathering, and chemicals.
- Neoprene seals are suitable for applications where abrasion resistance is a concern.
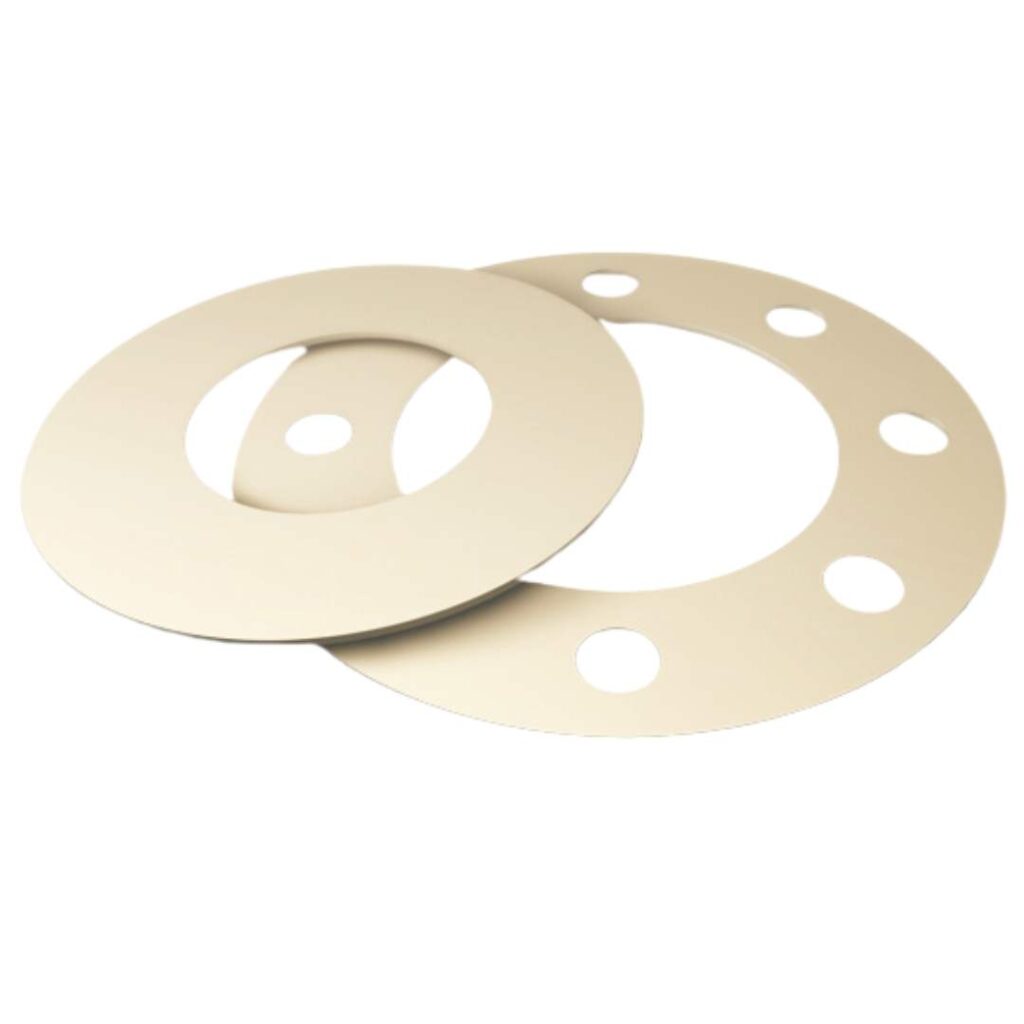
Nitrile (Buna-N) Seals:
- Nitrile rubber is widely used in seals due to its excellent oil resistance and flexibility.
- Nitrile seals are suitable for applications where exposure to oils and greases is a consideration.
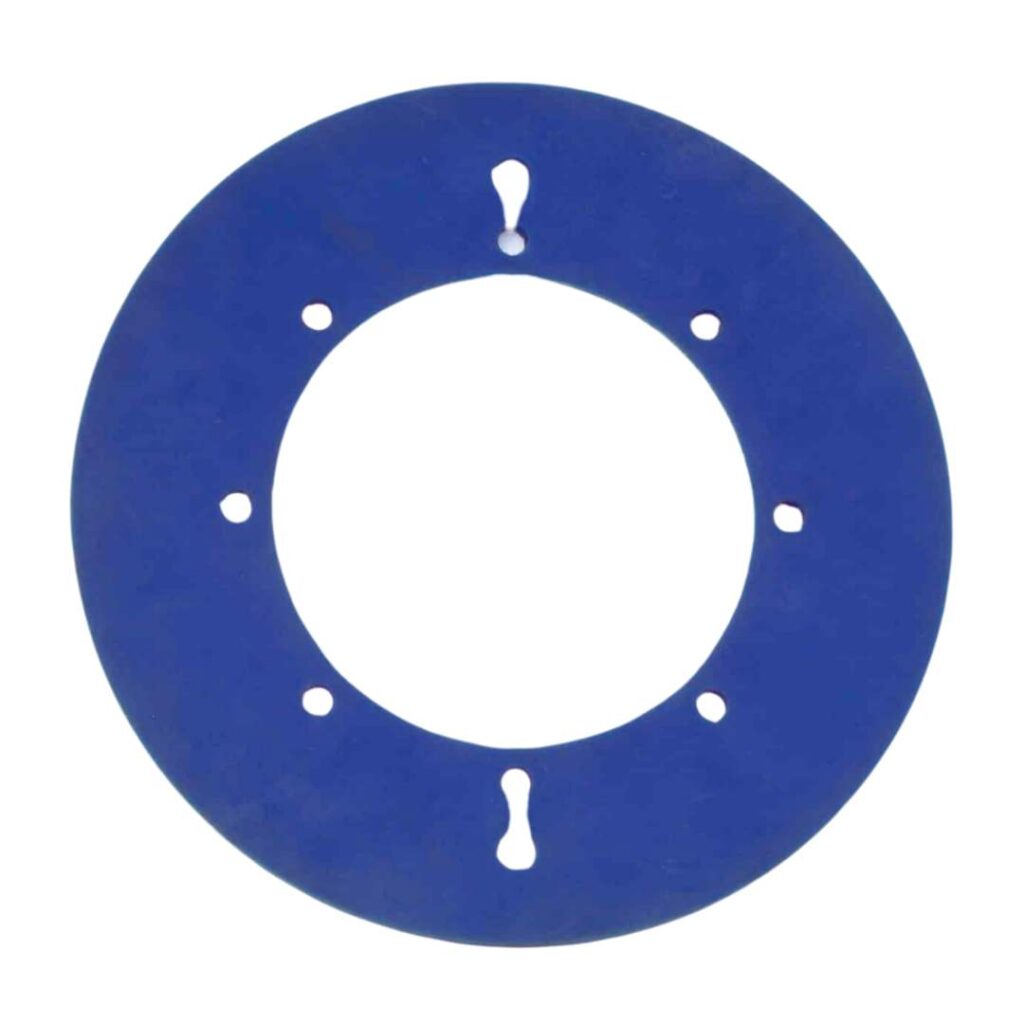
Polyurethane Seals:
- Polyurethane offers good abrasion resistance and durability.
- Polyurethane seals are suitable for applications involving abrasive materials and where extended wear life is required.
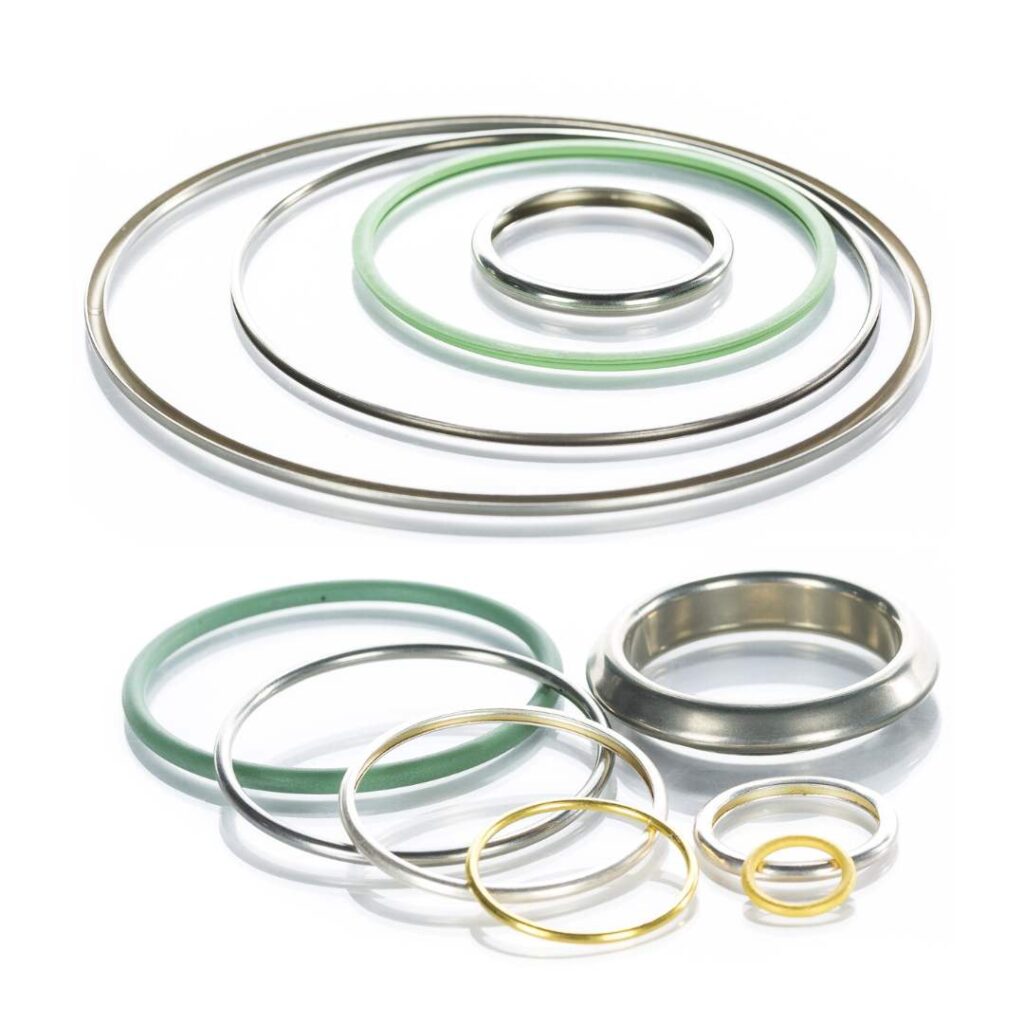
Metal-to-Metal Seals:
- In some high-temperature or high-pressure applications, metal-to-metal seals may be used.
- These seals are more robust and can withstand harsh operating conditions.
The selection of a specific seal depends on the specific requirements of the application, including the type of material being handled, the operating temperature and pressure, and the desired level of sealing effectiveness. Regular maintenance and monitoring of seals are important to ensure the continued reliability and performance of rotary airlock valves.
Types of Rotors used in Rotary Airlock Valve
There are several types of rotors used in rotary airlock valves, each designed for specific applications. The common types of rotors include:
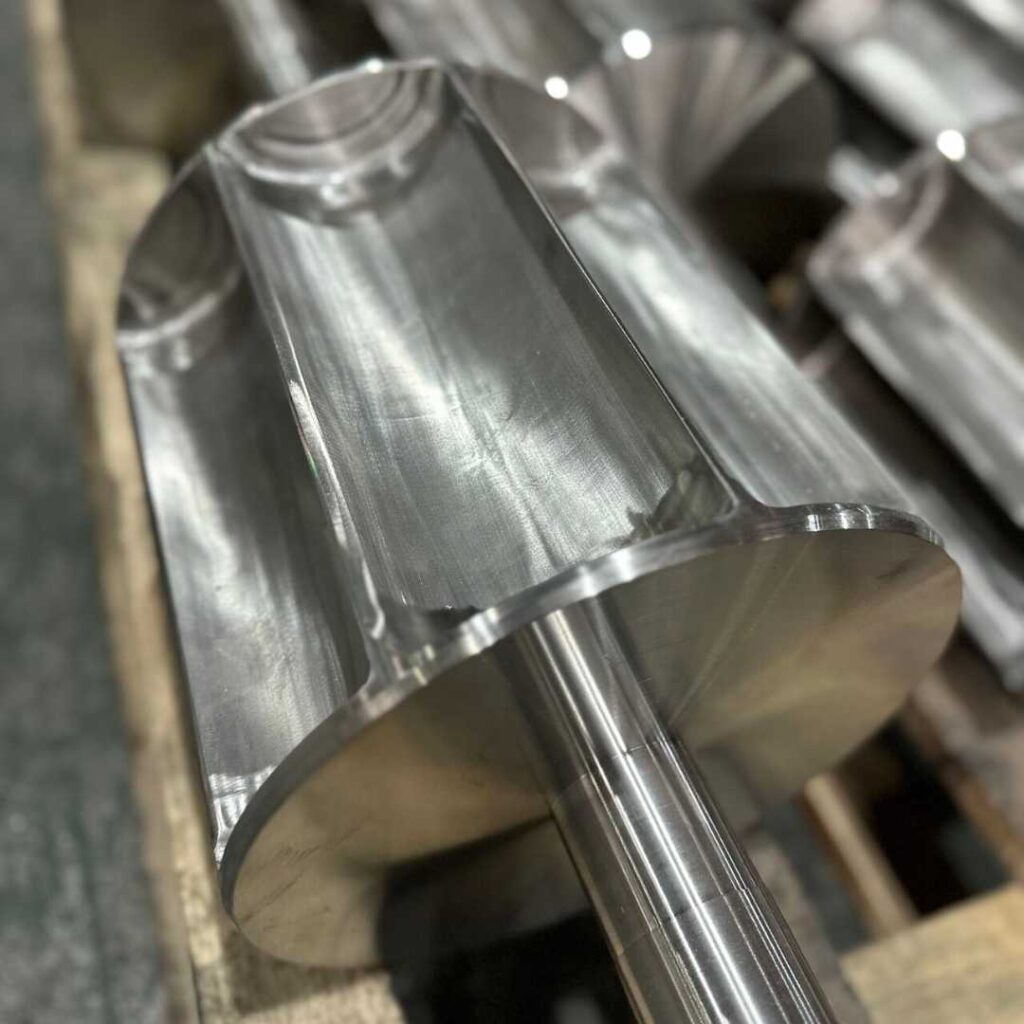
Closed-End Rotor
A closed-end rotor has solid ends on both sides. It is suitable for handling non-abrasive materials and applications where a tight seal is essential to prevent air leakage between different pressure zones.
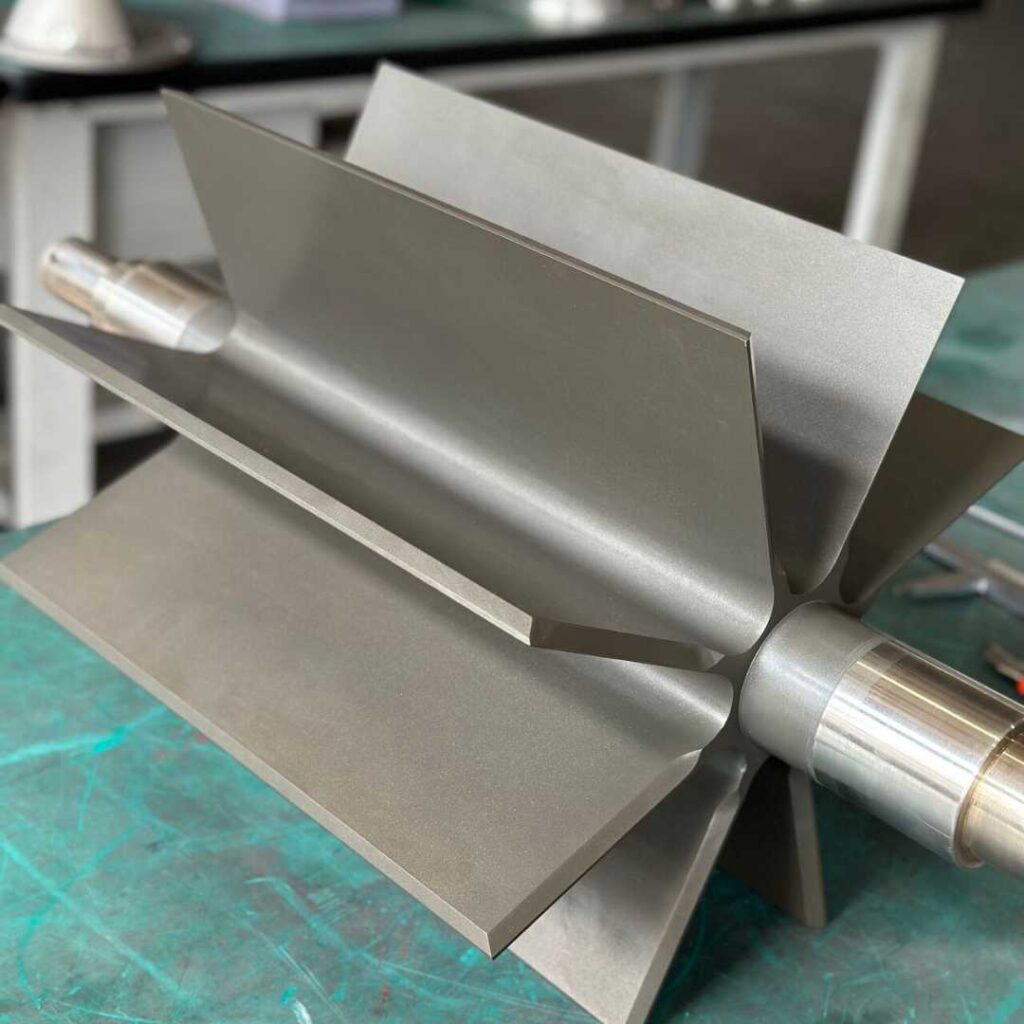
Open-End Rotor
An open-end rotor has one side open, allowing for easier material entry and discharge. It is commonly used in applications where material characteristics or system requirements permit some air leakage.
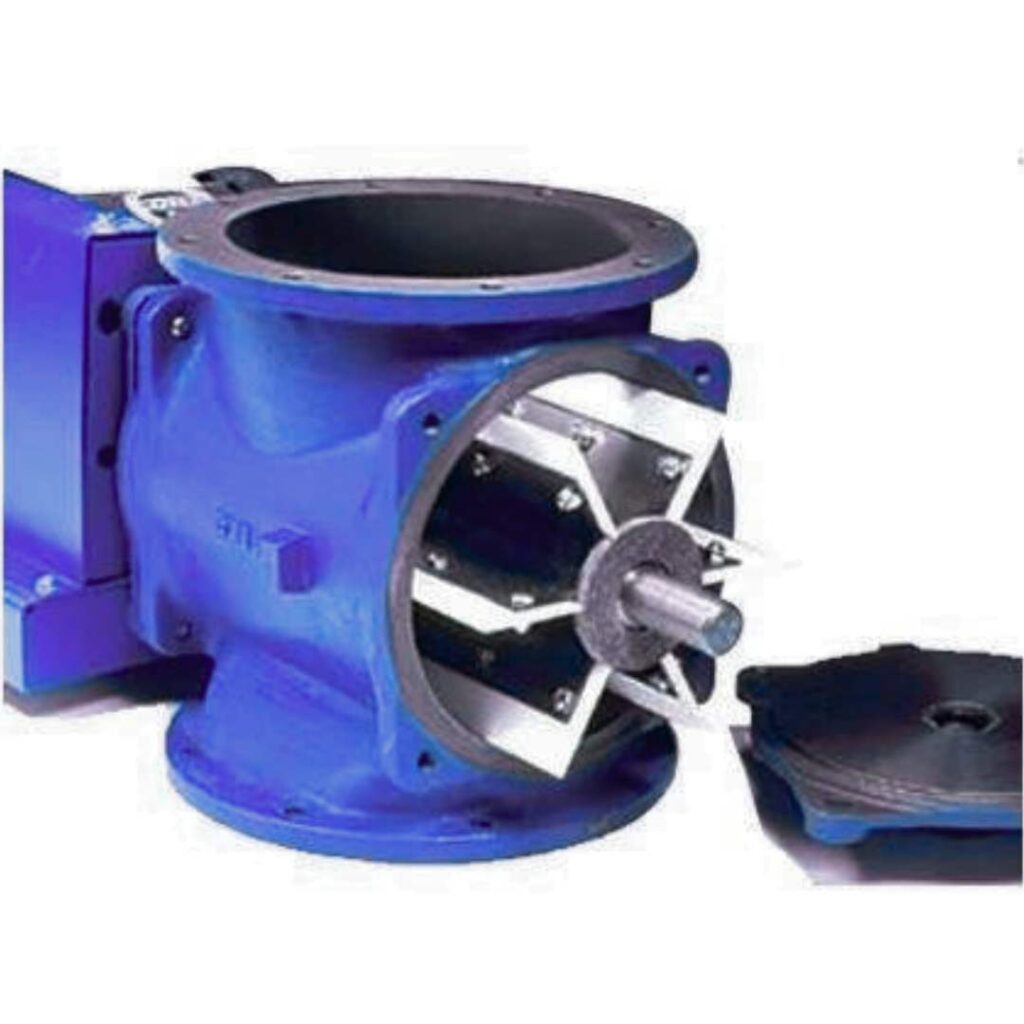
Dust-Collector Rotor
This type of rotor is designed with large pockets to collect and discharge fine dust or powders. It helps prevent material build-up and reduces the risk of rotor jamming, making it suitable for applications with dusty materials.
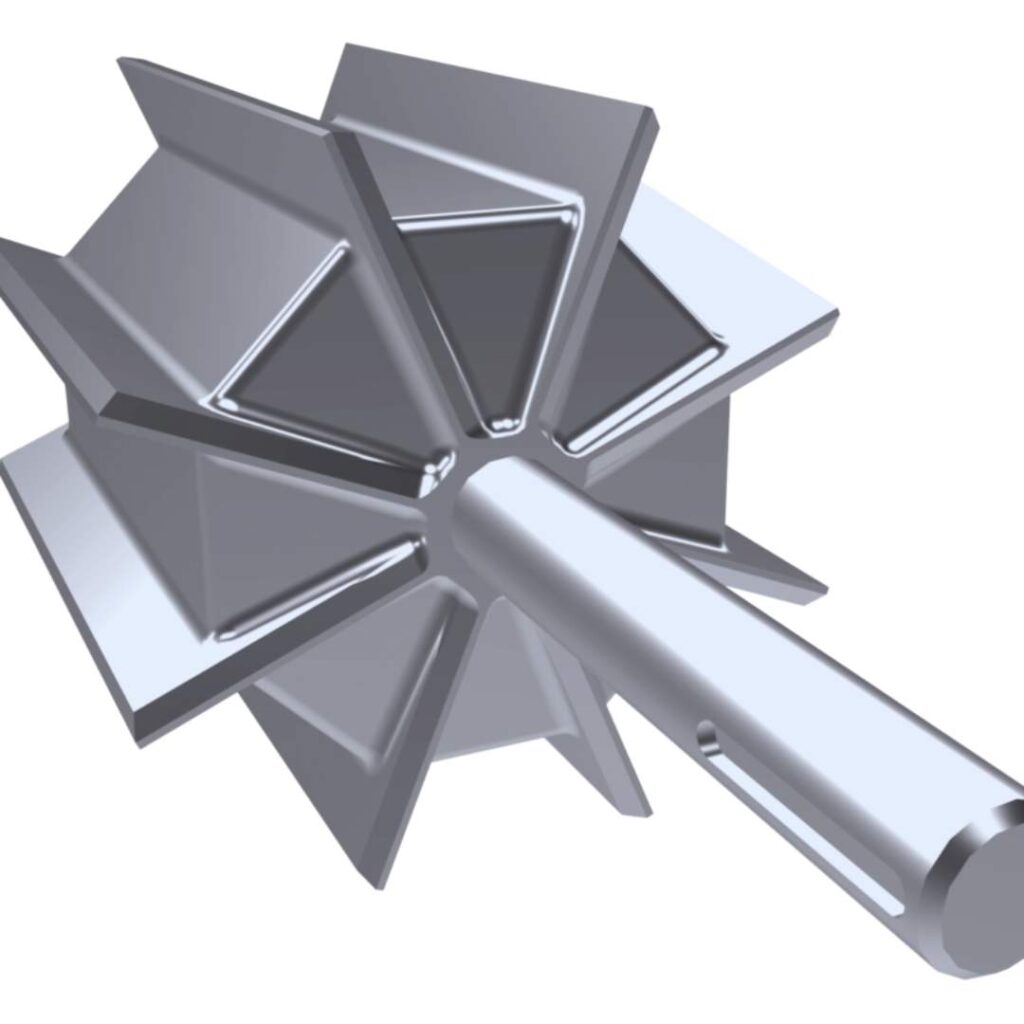
Shallow-Pocket Rotor:
Shallow-pocket rotors are designed with smaller pocket depths. They are often used for applications involving sticky or tacky materials to prevent material build-up within the pockets.
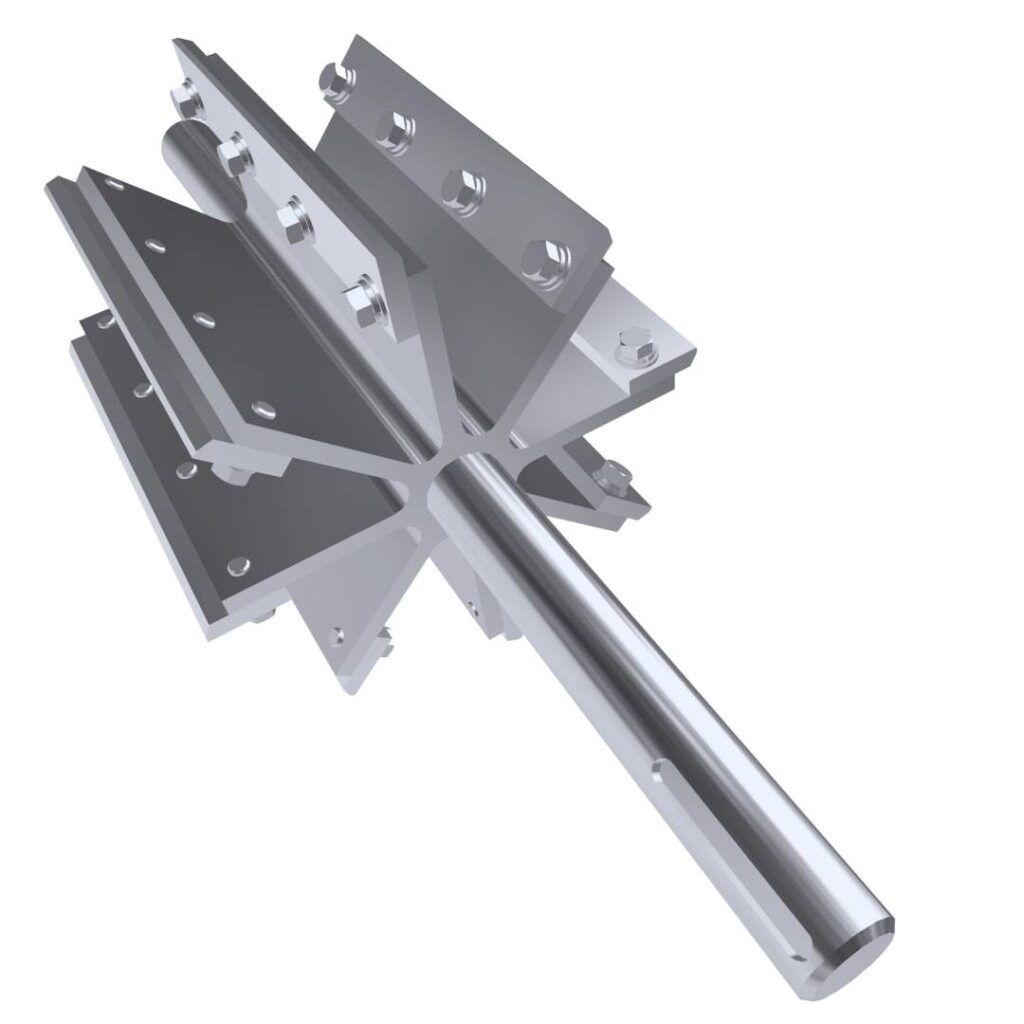
Adjustable-Tip Rotor:
Adjustable-tip rotors allow the rotor tips to be adjusted to maintain the proper clearance between the rotor and the housing. This feature is useful for fine-tuning the valve’s performance and addressing wear over time.
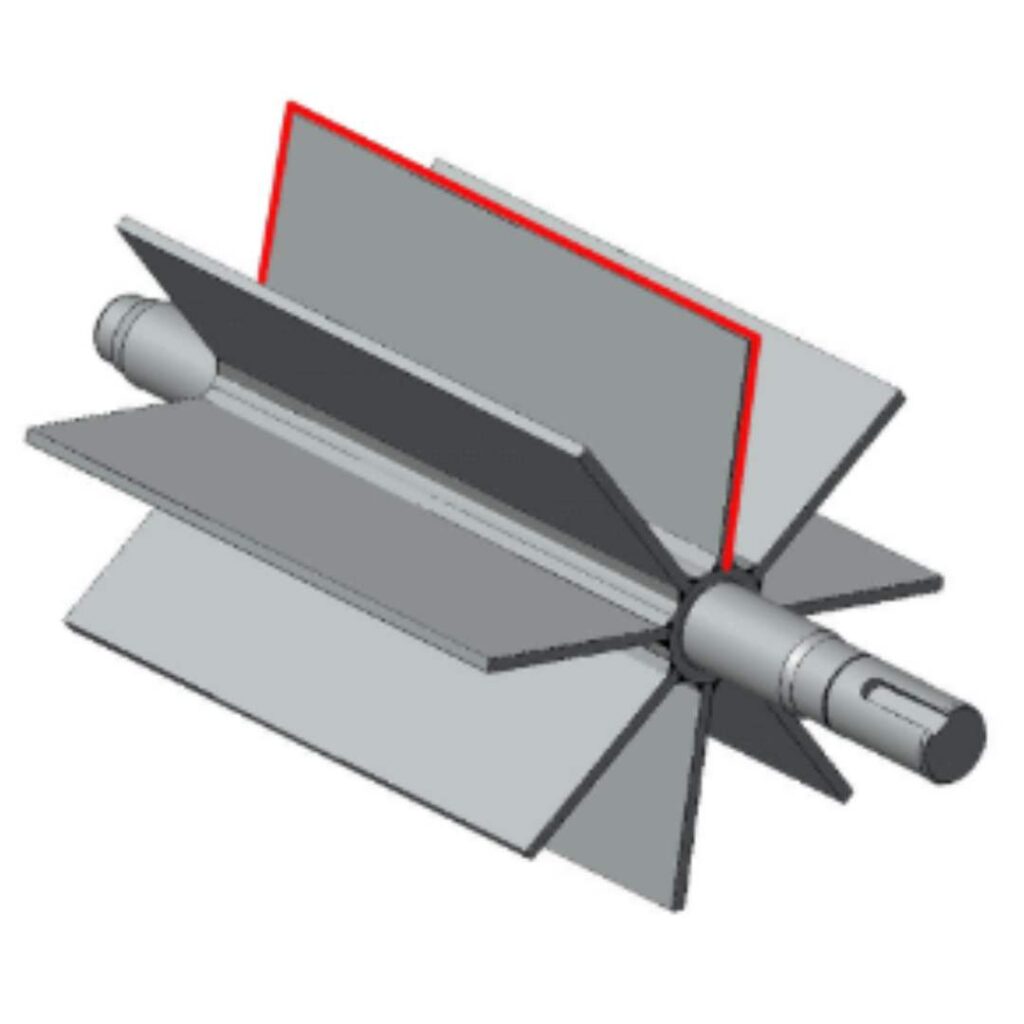
Rigid Pocket Rotor
Rigid pocket rotors have reinforced pockets, making them suitable for handling abrasive materials. They provide increased durability and resistance to wear.
Materials used for manufacturing Rotary Airlock Valve
Rotary airlock valves are crucial components in pneumatic conveying systems, and their construction materials are chosen based on factors such as the type of material being handled, the system’s operating conditions, and considerations for wear resistance. Common materials used for manufacturing rotary airlock valves include:
Carbon Steel:
Carbon steel is often used for the construction of rotary airlock valve components, including the endplates and rotor. It offers strength and durability in various operating environments.
Cast Iron:
Cast iron is a common material for rotary airlock valve housings and endplates. It provides good strength and wear resistance. Gray iron and ductile iron are variations of cast iron used in different applications.
Stainless Steel:
Stainless steel is chosen for applications where corrosion resistance is critical. Types such as 304 and 316 stainless steel are commonly used to prevent rust and corrosion, making them suitable for handling corrosive materials or operating in harsh environments.
Aluminum:
Aluminum is chosen for applications where weight is a significant consideration. It is lighter than steel, making it suitable for certain industries or applications with specific requirements.
Hardened Steel or Tool Steel:
Hardened or tool steel may be used for rotors, especially in applications where abrasion resistance is crucial. Hardened steel helps extend the life of the rotor in handling abrasive materials.
Ceramic Coatings:
Some rotary airlock valves may have ceramic coatings or inserts to enhance wear resistance, particularly when handling abrasive materials. Ceramic materials provide excellent resistance to abrasion.
Specialized Coatings:
Various specialized coatings, such as tungsten carbide, may be applied to critical wear areas to improve durability and extend the life of the rotary airlock valve in challenging conditions.
Polymer and Composite Materials:
In certain applications, polymer or composite materials may be used for specific components to provide chemical resistance or reduce wear. This can include polymer housings or coatings.
Factors to be considered for selection of Rotary Airlock Valve
Material to be conveyed
When choosing a rotary airlock, consider the material characteristics such as abrasiveness, moisture content, and particle size. Selection factors include using hard-faced components for abrasives and ensuring a tight seal for moist materials to prevent air entry and drying. The rotary airlock should match the specific requirements of the material and its flow characteristics.
Flow Rate
For effective pneumatic conveying systems, choose a rotary airlock that matches the material’s flow rate. Avoid bottlenecks by ensuring the rotor’s size and speed align with the flow rate. An appropriately sized rotary airlock enhances energy efficiency and reduces maintenance costs.
Pressure Drop
When selecting a rotary airlock, be mindful of pressure drop—the difference between inlet and outlet pressures. High pressure drop reduces system efficiency and increases energy costs. To minimize this, choose a rotary airlock with a tight seal and a rotor correctly sized for the flow rate.
Type of Pneumatic Conveying System
Selecting the right rotary airlock depends on the type of pneumatic conveying system. Dilute phase systems benefit from flow-through rotary airlocks (drop-through), suitable for materials like grain, coffee beans, polymer granules, etc. Materials like flour, cocoa powder, chemical powders work well with blow-through rotary airlocks. Matching the rotary airlock to the conveying system ensures optimal performance.
Maintenance and Repair
Go for designs that provide convenient access to the rotor and vanes. Look for rotary airlocks with replaceable parts to minimize downtime and lower maintenance costs.